genes
Snapshots of Life: Fish Awash in Color
Posted on by Dr. Francis Collins

If this image makes you think of a modern art, you’re not alone. But what you’re actually seeing are hundreds of live cells from a tiny bit (0.0003348 square inches) of skin on the tail fin of a genetically engineered adult zebrafish. Zebrafish are normally found in tropical freshwater and are a favorite research model to study vertebrate development and tissue regeneration. The cells have been labeled with a cool, new fluorescent imaging tool called Skinbow. It uniquely color codes cells by getting them to express genes encoding red, green, and blue fluorescent proteins at levels that are randomly determined. The different ratios of these colorful proteins mix to give each cell a distinctive hue when imaged under a microscope. Here, you can see more than 70 detectable Skinbow colors that make individual cells as visually distinct from one another as jellybeans in a jar.
Skinbow is the creation of NIH-supported scientists Chen-Hui Chen and Kenneth Poss at Duke University, Durham, NC, with imaging computational help from collaborators Stefano Di Talia and Alberto Puliafito. As reported recently in the journal Developmental Cell [1], Skinbow’s distinctive spectrum of color occurs primarily in the outermost part of the skin in a layer of non-dividing epithelial cells. Using Skinbow, Poss and colleagues tracked these epithelial cells, individually and as a group, over their entire 2 to 3 week lifespans in the zebrafish. This gave them an unprecedented opportunity to track the cellular dynamics of wound healing or the regeneration of lost tissue over time. While Skinbow only works in zebrafish for now, in theory, it could be adapted to mice and maybe even humans to study skin and possibly other organs.
Study Shows DNA Sequencing Brings Greater Precision to Childhood Cancer
Posted on by Dr. Francis Collins
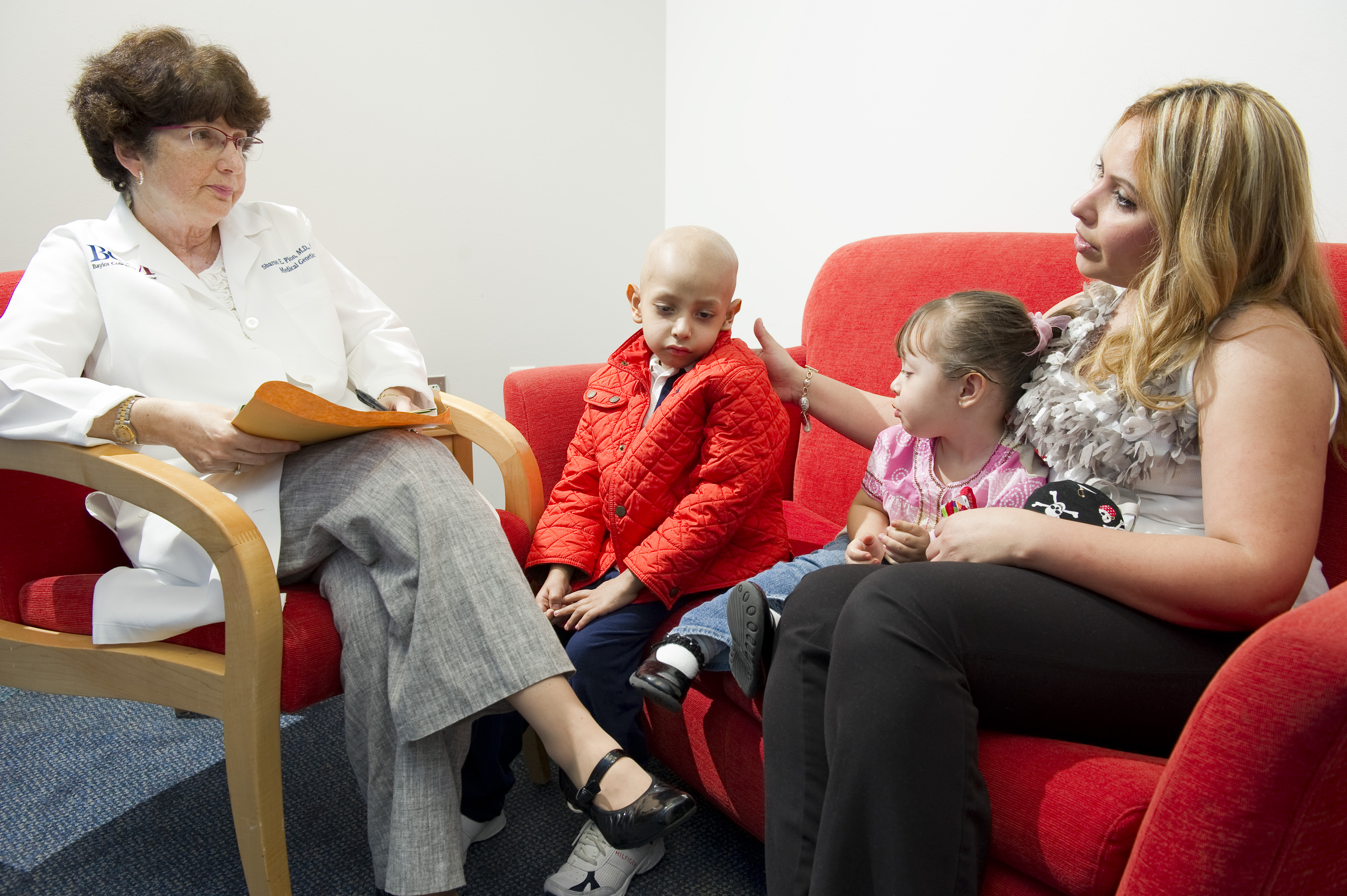
Caption: Baylor’s Sharon Plon consults with a family at the Texas Children’s Cancer Center in Houston.
Credit: Paul V. Kuntz/Texas Children’s Hospital
An impressive number of fundamental advances in our understanding of cancer have occurred over the past several decades. One of the most profound is the realization that cancer is a disease of the genome, driven by a wide array of changes in DNA—some in the germline and affecting all cells of the body, but most occurring in individual cells during life (so-called “somatic mutations”). As the technology for sequencing cancer genomes has advanced, we are learning that virtually all cancers carry a unique set of mutations. Most are DNA copying errors of no significance (we call those “passengers”), but a few of them occur in genes that regulate cell growth and contribute causatively to the cancer (we call those “drivers”). We are now learning that it may be far more important for treating cancer to figure out what driver mutations are present in a patient’s tumor than to identify in which organ it arose. And, as a new study shows, this approach even appears to have potential to help cancer’s littlest victims.
Using genomic technology to analyze both tumor and blood samples from a large number of children who’d been newly diagnosed with cancer, an NIH-funded research team uncovered genetic clues with the potential to refine diagnosis, identify inherited cancer susceptibility, or guide treatment for nearly 40 percent of the children [1]. The potential driver mutations spanned a broad spectrum of genes previously implicated not only in pediatric cancers, but also in adult cancers. While much more work remains to determine how genomic analyses can be used to devise precise, new strategies for treating kids with cancer, the study provides an excellent example of the kind of research that NIH hopes to accelerate under the nation’s new cancer “moonshot,” a research initiative recently announced by the President and being led by the Vice President.
Autism Architecture: Unrolling the Genetic Blueprint
Posted on by Dr. Francis Collins
We know that a combination of genetic and environmental factors influence a child’s risk of autism spectrum disorder (ASD), which is a diverse group of developmental brain conditions that disrupt language, communication, and social interaction. Still, there remain a great many unknowns, including the crucial issues of what proportion of ASD risk is due to genes and what sorts of genes are involved. Answering such questions may hold the key to expanding our understanding of the disorder—and thereby to devising better ways to help the millions of Americans whose lives are touched by ASD [1].
Last year, I shared how NIH-funded researchers had identified rare, spontaneous genetic mutations that appear to play a role in causing ASD. Now, there’s additional news to report. In the largest study of its kind to date, an international team supported by NIH recently discovered that common, inherited genetic variants, acting in tandem with each other or with rarer variants, can also set the stage for ASD—accounting for nearly half of the risk for what’s called “strictly defined autism,” the full-blown manifestation of the disorder. And, when the effects of both rare and common genetic variants are tallied up, we can now trace about 50 to 60 percent of the risk of strictly defined autism to genetic factors.
Mining the Big Data Mountain
Posted on by Dr. Francis Collins
Biomedical researchers and clinicians are generating an enormous, ever-expanding trove of digital data through DNA sequencing, biomedical imaging, and by replacing a patient’s medical chart with a lifelong electronic medical record. What can be done with all of this “Big Data”?
Besides being handy for patients and doctors, Big Data may provide priceless raw material for the next era of biomedical research. Today, I want to share an example of research that is leveraging the power of Big Data.
Exploring the Complex Genetics of Schizophrenia
Posted on by Dr. Francis Collins
Schizophrenia is one of the most prevalent, tragic, and frustrating of all human illnesses, affecting about 1% of the human population, or 2.4 million Americans [1]. Decades of research have failed to provide a clear cause in most cases, but family clustering has suggested that inheritance must play some role. Over the last five years, multiple research projects known as genome-wide association studies (GWAS) have identified dozens of common variations in the human genome associated with increased risk of schizophrenia [2]. However, the individual effects of these variants are weak, and it’s often not been clear which genes were actually affected by the variations. Now, advances in DNA sequencing technology have made it possible to move beyond these association studies to study the actual DNA sequence of the protein-coding region of the entire genome for thousands of individuals with schizophrenia. Reports just published have revealed a complex constellation of rare mutations that point to specific genes—at least in certain cases.
siRNAs: Small Molecules that Pack a Big Punch
Posted on by Dr. Francis Collins

Credit: Richard J. Youle Laboratory, NINDS, NIH
It would be terrific if we could turn off human genes in the laboratory, one at a time, to figure out their exact functions and learn more about how our health is affected when those functions are disrupted. Today, I’m excited to announce the availability of new data that will empower researchers to do just that on a genome-wide scale. As part of a public-private collaboration between the NIH’s National Center for Advancing Translational Sciences (NCATS) and Life Technologies Corporation, researchers now have access to a wealth of information about small interfering RNAs (siRNAs), which are snippets of ribonucleic acid (RNA) with the power to turn off a gene, or reduce its activity—in much the same way that we use a dimmer switch to modulate a light.
Snapshots of Life: Development on Display
Posted on by Dr. Francis Collins
What on earth is this strange-looking critter? Well, among other things, it’s a scientific super model whose photo shoot landed it among the winners of the Federation of American Societies for Experimental Biology’s 2013 BioArt Competition. Researchers use this stingray-like sea creature, called Leucoraja erinacea or Little Skate, as a model organism for studying development.
This image, taken using a stereomicroscope with transmitted light, shows a 10-week-old Little Skate embryo attached to its nutrient-rich yolk sac. Because the skate can develop normally even when removed from its egg case, it provides an accessible system for exploring how genes direct the formation of internal organs.
The diversity found in the natural world can also reveal unexpected insights into human disease. For example, it turns out that the genes controlling development of the Little Skate’s fins are strongly influenced by male sex hormones. And this is the really surprising part: researchers have discovered that the genes activated in the skate fins are the same genes that respond to hormones in human prostate, breast, and skin cancers. So, by studying these genes in this bizarre-looking denizen of the deep, it’s possible to probe the genes that trigger disease in humans.
Links:
BioArt, Federation of American Societies for Experimental Biology
Martin Cohn, Molecular Genetics & Microbiology, University of Florida
BioArt 2013 Exhibit. The public can view an exhibit of the winning art at the NIH Visitor Center. Located in Bethesda, MD, the Center is open from 8:30 a.m.–4:30 p.m. M–F.
NIH support: National Institute of Environmental Health Sciences; National Institute of Diabetes and Digestive and Kidney Diseases
Network News: Gene Discoveries for Autism
Posted on by Dr. Francis Collins
Affecting an estimated 1 in 88 U.S. children, autism spectrum disorder (ASD) is a complicated and diverse group of developmental brain disorders that interfere with language, normal communication, and social interaction. Unlike some other conditions that are caused by mutations in a single gene, as many as 1,000 genes, as well as various environmental factors, are suspected to contribute to the risk of developing ASD. That’s daunting because before we can develop broadly-applicable treatments, we need to figure out which are the key genes, what brain cells they control, and when they are active.
Fighting Obesity: New Hopes From Brown Fat
Posted on by Dr. Francis Collins

Illustration: John MacNeill, based on patient imaging software designed by Ilan Tal. Copyright 2011 Joslin Diabetes Center
If you want to lose weight, then you actually want more fat, not less. But you need the right kind: brown fat. This special type of fatty tissue burns calories, puts out heat like a furnace, and helps to keep you trim. White fat, on the other hand, stores extra calories and makes you, well, fat. Wouldn’t it be nice if we could instruct our bodies to make more brown fat, and less white fat? Well, NIH-funded researchers have just taken another step in that direction [1].
New Understanding of a Common Kidney Cancer
Posted on by Dr. Francis Collins

Caption: Histologic image of clear cell kidney cancer
Slide courtesy of W. Marston Linehan, National Cancer Institute, NIH
Understanding how cancer cells shift into high gear—what makes them become more aggressive and unresponsive to treatment—is a key concern of cancer researchers. A new study reveals how this escalation occurs in the most common form of kidney cancer: clear cell renal cell carcinoma (ccRCC). The study shows that ccRCC tumors acquire specific mutations that encourage uncontrollable growth and shifts in energy use and production [1].
Conducted by researchers in the NIH-led The Cancer Genome Atlas (TCGA) Research Network, the study compared more than 400 ccRCC tumors from individual patients with healthy tissue samples from the same patients. Researchers were looking for differences in the gene activity and proteins in healthy vs. tumor tissue.
Previous Page Next Page