sleep
Study Suggests During Sleep, Neural Process Helps Clear the Brain of Damaging Waste
Posted on by Dr. Monica M. Bertagnolli
We’ve long known that sleep is a restorative process necessary for good health. Research has also shown that the accumulation of waste products in the brain is a leading cause of numerous neurological disorders, including Alzheimer’s and Parkinson’s diseases. What hasn’t been clear is how the healthy brain “self-cleans,” or flushes out that detrimental waste.
But a new study by a research team supported in part by NIH suggests that a neural process that happens while we sleep helps cleanse the brain, leading us to wake up feeling rested and restored. Better understanding this process could one day lead to methods that help people function well on less sleep. It could also help researchers find potential ways to delay or prevent neurological diseases related to accumulated waste products in the brain.
The findings, reported in Nature, show that, during sleep, neural networks in the brain act like an array of miniature pumps, producing large and rhythmic waves through synchronous bursts of activity that propel fluids through brain tissue. Much like the process of washing dishes, where you use a rhythmic motion of varying speeds and intensity to clear off debris, this process that takes place during sleep clears accumulated metabolic waste products out.
The research team, led by Jonathan Kipnis and Li-Feng Jiang-Xie at Washington University School of Medicine in St. Louis, wanted to better understand how the brain manages its waste. This is not an easy task, given that the human brain’s billions of neurons inevitably produce plenty of junk during cognitive processes that allow us to think, feel, move, and solve problems. Those waste products also build in a complex environment, including a packed maze of interconnected neurons, blood vessels, and interstitial spaces, surrounded by a protective blood-brain barrier that limits movement of substances in or out.
So, how does the brain move fluid through those tight spaces with the force required to get waste out? Earlier research suggested that neural activity during sleep might play an important role in those waste-clearing dynamics. But previous studies hadn’t pinned down the way this works.
To learn more in the new study, the researchers recorded brain activity in mice. They also used an ultrathin silicon probe to measure fluid dynamics in the brain’s interstitial spaces. In awake mice, they saw irregular neural activity and only minor fluctuations in the interstitial spaces. But when the animals were resting under anesthesia, the researchers saw a big change. Brain recordings showed strongly enhanced neural activity, with two distinct but tightly coupled rhythms. The research team realized that the structured wave patterns could generate strong energy that could move small molecules and peptides, or waste products, through the tight spaces within brain tissue.
To make sure that the fluid dynamics were really driven by neurons, the researchers used tools that allowed them to turn neural activity off in some areas. Those experiments showed that, when neurons stopped firing, the waves also stopped. They went on to show similar dynamics during natural sleep in the animals and confirmed that disrupting these neuron-driven fluid dynamics impaired the brain’s ability to clear out waste.
These findings highlight the importance of this cleansing process during sleep for brain health. The researchers now want to better understand how specific patterns and variations in those brain waves lead to changes in fluid movement and waste clearance. This could help researchers eventually find ways to speed up the removal of damaging waste, potentially preventing or delaying certain neurological diseases and allowing people to need less sleep.
Reference:
[1] Jiang-Xie LF, et al. Neuronal dynamics direct cerebrospinal fluid perfusion and brain clearance. Nature. DOI: 10.1038/s41586-024-07108-6 (2024).
NIH Support: National Center for Complementary and Integrative Health
What’s Behind that Morning Migraine? Community-Based Study Points to Differences in Perceived Sleep Quality, Energy on the Previous Day
Posted on by Dr. Monica M. Bertagnolli
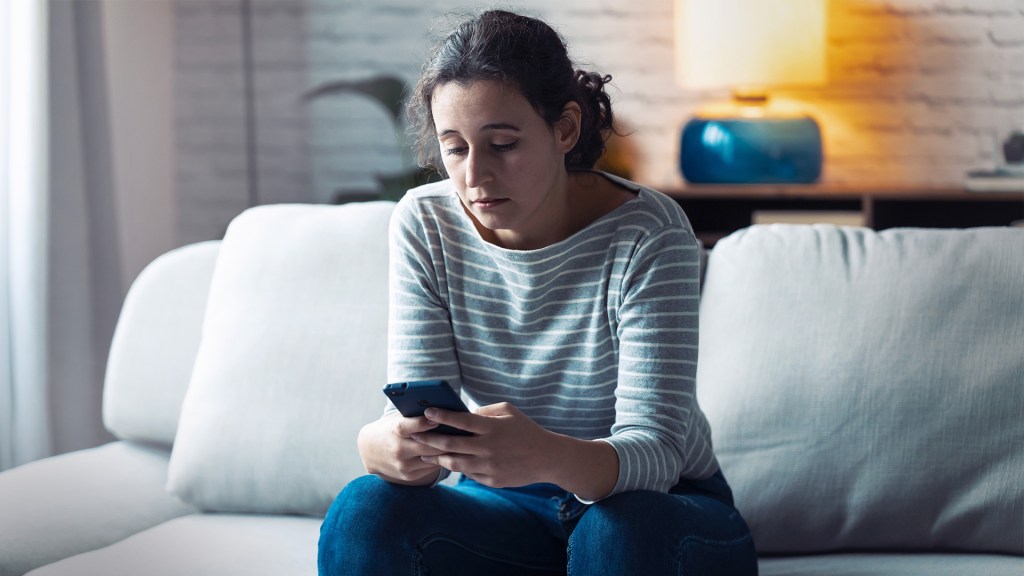
Headaches are the most common form of pain and a major reason people miss work or school. Recurrent attacks of migraine headaches can be especially debilitating, involving moderate to severe throbbing and pulsating pain on one side of the head that sometimes lasts for days. Migraines and severe headaches affect about 1 in 5 women and about 1 in 10 men, making them one of the most prevalent of all neurological disorders.1 And yet there’s still a lot we don’t know about what causes headaches or how to predict when one is about to strike.
Now a new NIH-led study reported in the journal Neurology has some important insight.2 One of the things I especially appreciate about this new work is that it was conducted in a community setting rather than through a specialty clinic, with people tracking their own headache symptoms, sleep, mood, and more on a mobile phone app while they went about their daily lives. It means that the findings are extremely relevant to the average migraine sufferer who shows up in a primary care doctor’s office looking for help for their recurrent headaches.
The study, led by Kathleen Merikangas at NIH’s National Institute of Mental Health, Bethesda, MD, is part of a larger, community-based Family Study of Affective and Anxiety Spectrum Disorders. This ongoing study enrolls volunteers from the greater Washington, D.C., area with a range of disorders, including bipolar disorders, major depression, anxiety disorders, sleep disorders, and migraine along with their immediate family members. It also includes people with none of these disorders who serve as a control group. The goal is to learn more about the frequency of mood and other mental and physical disorders in families and how often they co-occur. This information can provide insight into the nature and causes for all these conditions.
While there will be much more to come from this ambitious work, the primary aim for this latest study was to look for links between a person’s perceived mood, sleep, energy, and stress and their likelihood for developing a headache. The study’s 477 participants, aged 7 to 84, included people with and without migraines who were also assessed for mood, anxiety, sleep disorders and other physical conditions. Women accounted for 291 of the study’s participants. Each were asked to track their emotional states, including anxiousness, mood, energy, stress, and headaches four times each day for two weeks. Each morning, they also reported on their sleep the night before.
The data showed that people with a morning migraine reported poorer quality sleep the night before. They also reported lower energy the day before. Interestingly, those factors didn’t lead to an increased risk of headaches in the afternoon or evening. Afternoon or evening headaches were more often preceded by higher stress levels or having higher-than-average energy the day before.
More specifically, people with poorer perceived sleep quality on average had a 22 percent greater chance for a headache attack the next morning. A decrease in the self-reported usual quality of sleep was also associated with an 18 percent increased chance of a headache the next morning. Similarly, a drop in the usual level of energy on the prior day was associated with a 16 percent greater chance of headache the next morning. In contrast, greater average levels of stress and substantially higher energy than usual the day before was associated with a 17 percent increased chance of headache later the next day.
Surprisingly, the study didn’t find any connection between feeling anxious or depressed with headaches on the next day after considering energy and sleep. However, Merikangas emphasizes that participants’ perceived differences in energy and sleep may not reflect objective measures of sleep patterns or energy, suggesting that the connection may still be based on changes in a person’s feelings about their underlying physical or emotional state in complex ways.
The findings suggest that changes in the body and brain are already taking place before a person first feels a headache, suggesting it may be possible to predict and prevent migraines or other headaches. It also adds to evidence for the usefulness of diaries or apps for headache sufferers to track their sleep, health, behavioral, and emotional states in real time to better understand and manage headache pain. Meanwhile, the researchers report that they’re continuing to explore other factors that may precede and trigger headaches, including dietary factors, changes in a person’s physiology such as stress hormone levels, and environmental factors, including weather, seasonal changes, and geography.
References:
[1] American Headache Society. The Prevalence of Migraine and Severe Headache.
[2] Lateef TM, et al. Association Between Electronic Diary-Rated Sleep, Mood, Energy, and Stress With Incident Headache in a Community-Based Sample. Neurology. DOI: 10.1212/WNL.0000000000208102. (2024).
NIH Support: National Institute of Mental Health
Small Study Suggests Approved Insomnia Drug Can Aid in Opioid Recovery
Posted on by Lawrence Tabak, D.D.S., Ph.D.

Opioid use disorders (OUD) now threaten the health and lives of far too many young and adult Americans. While getting treatment is a key first step to recovery, overcoming an opioid addiction often comes with brutal withdrawal symptoms, including bad bouts of insomnia that are often untreatable with traditional prescription sleep medications. These medications act as sedatives, making them unsafe for people in OUD recovery.
But now, researchers have found that an approved drug for insomnia that works differently than other sleep medications could offer some needed help for the sleeplessness that affects those overcoming an opioid addiction [1]. The drug, known as suvorexant (Belsomra ®), was provided in a study to people during and immediately after tapering off opioids, and it allowed them to sleep significantly more during this week-long period. Suvorexant also helped to reduce their opioid withdrawal and craving.
This study, which received support from NIH’s Helping to End Addiction Long-term (HEAL) Initiative certainly offers promising news. The Food and Drug Administration (FDA) approved suvorexant to treat insomnia in 2014, and it is available for off-label use to help people overcoming an OUD.
The good news, however, comes with a major caveat. This early clinical trial had relatively small enrollment numbers, and larger studies are definitely needed to follow up and confirm the initial results.
The latest findings, published in the journal Science Translational Medicine, come from a team at Johns Hopkins University School of Medicine, Baltimore, led by Andrew Huhn. He and colleagues recognized sleep disturbances as a severe problem during recovery. They wondered whether suvorexant might help.
Suvorexant doesn’t actively sedate people like other sleeping medications. Suvorexant works by targeting orexin, a biochemical made in the brain that helps keep you awake [2]. Interestingly, orexin signals also have been implicated in opioid withdrawal symptoms, sleep disturbances, and drug-seeking behaviors.
Thirty-eight people entered the Hopkins study, and 26 completed it. Their average age was about 40, with close to equal numbers of white and Black participants. Most were male, and all were undergoing supervised withdrawal treatment with buprenorphine/naloxone, which is used in combination as a medication-assisted treatment for OUD.
To find out if suvorexant helped, the researchers measured total sleep time nightly using wireless devices that recorded brain activity and movement in people taking either 20 milligrams or 40 milligrams of suvorexant versus a placebo. The researchers also used standard methods to assess symptoms of opioid withdrawal, along with suvorexant’s potential for abuse.
The data showed that people taking suvorexant over four days while tapering off opioids slept about 90 minutes longer per night on average. They also continued to sleep for an extra hour a night on average in the four days following the tapering period. The researchers note that these increases in sleep duration far exceed the American Academy of Sleep Medicine’s threshold for clinically meaningful improvement.
The researchers also didn’t see any differences in adverse events between those taking suvorexant versus a placebo. They also note that the main side effect of suvorexant in general is feeling sleepy the next day as the drug wears off slowly. There also wasn’t any evidence that suvorexant might come with a risk for drug abuse.
However, because the study was small, it lacked the needed statistical power to determine meaningful differences between the two doses of suvorexant. The study also didn’t include many women. But overall, the evidence that suvorexant or even other medications that target orexin could improve OUD treatment appears quite promising.
The NIH’s HEAL Initiative has launched over 600 research projects across the country. These studies cover a range of science and health care needs. But a common thread running through these projects is a desire to enhance the evidence base for lifesaving OUD interventions. Another is a commitment to discover better ways to help people recover from an OUD, and these latest data on suvorexant show this commitment in action.
References:
[1] Suvorexant ameliorated sleep disturbance, opioid withdrawal, and craving during a buprenorphine taper. Huhn AS, Finan PH, Gamaldo CE, Hammond AS, Umbricht A, Bergeria CL, Strain EC, Dunn KE. Sci Transl Med. 2022 Jun 22;14(650):eabn8238.
[2] The hypocretin/orexin system. Ebrahim IO, et al. J R Soc Med. 2002 May;95(5):227-30.
Links:
SAMHSA’s National Helpline (Substance Abuse and Mental Health Services Administration, Rockville, MD)
Opioids (National Institute on Drug Abuse/NIH)
Helping to End Addiction Long-term (HEAL) Initiative (NIH)
Andrew Huhn (Johns Hopkins School of Medicine, Baltimore)
NIH Support: National Institute on Drug Abuse
Decoding Heart-Brain Talk to Prevent Sudden Cardiac Deaths
Posted on by Dr. Francis Collins
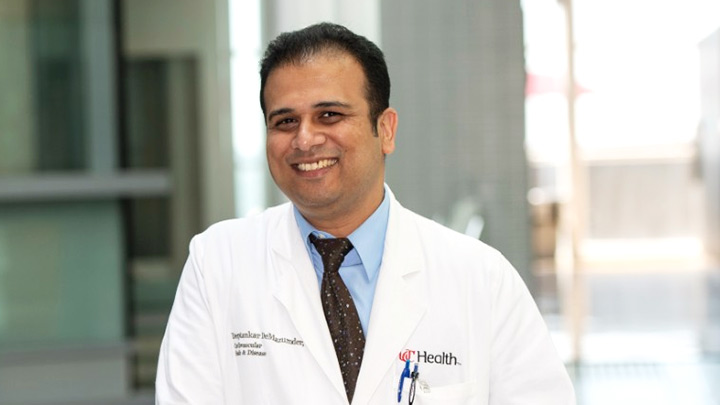
As a cardiac electrophysiologist, Deeptankar DeMazumder has worked for years with people at risk for sudden cardiac arrest (SCA). Despite the latest medical advances, less than 10 percent of individuals stricken with an SCA will survive this highly dangerous condition in which irregular heart rhythms, or arrhythmias, cause the heart suddenly to stop beating.
In his role as a physician, DeMazumder keeps a tight focus on the electrical activity in their hearts, doing his best to prevent this potentially fatal event. In his other role, as a scientist at the University of Cincinnati College of Medicine, DeMazumder is also driven by a life-saving aspiration: finding ways to identify at-risk individuals with much greater accuracy than currently possible—and to develop better ways of protecting them from SCAs. He recently received a 2020 NIH Director’s New Innovator Award to pursue one of his promising ideas.
SCAs happen without warning and can cause death within minutes. Poor heart function and abnormal heart rhythms are important risk factors, but it’s not possible today to predict reliably who will have an SCA. However, doctors already routinely capture a wealth of information in electrical signals from the heart using electrocardiograms (ECGs). They also frequently use electroencephalograms (EEGs) to capture electrical activity in the brain.
DeMazumder’s innovative leap is to look at these heart and brain signals jointly, as well as in new ways, during sleep. According to the physician-scientist, sleep is a good time to search for SCA signatures in the electrical crosstalk between the heart and the brain because many other aspects of brain activity quiet down. He also thinks it’s important to pay special attention to what happens to the body’s electrical signals during sleep because most sudden cardiac deaths happen early in the waking hours, for reasons that aren’t well understood.
He has promising preliminary evidence from both animal models and humans suggesting that signatures within heart and brain signals are unique predictors of sudden death, even in people who appear healthy [1]. DeMazumder has already begun developing a set of artificial intelligence algorithms for jointly deciphering waveform signals from the heart, brain, and other body signals [2,3]. These new algorithms associate the waveform signals with a wealth of information available in electronic health records to improve upon the algorithm’s ability to predict catastrophic illness.
DeMazumder credits his curiosity about what he calls the “art and science of healing” to his early childhood experiences and his family’s dedication to community service in India. It taught him to appreciate the human condition, and he has integrated this life-long awareness into his Western medical training and his growing interest in computer science.
For centuries, humans have talked about how true flourishing needs both head and heart. In DeMazumder’s view, science is just beginning to understand the central role of heart-brain conversations in our health. As he continues to capture and interpret these conversations through his NIH-supported work, he hopes to find ways to identify individuals who don’t appear to have serious heart disease but may nevertheless be at high risk for SCA. In the meantime, he will continue to do all he can for the patients in his care.
References:
[1] Mitochondrial ROS drive sudden cardiac death and chronic proteome remodeling in heart failure. Dey S, DeMazumder D, Sidor A, Foster DB, O’Rourke B. Circ Res. 2018;123(3):356-371.
[2] Entropy of cardiac repolarization predicts ventricular arrhythmias and mortality in patients receiving an implantable cardioverter-defibrillator for primary prevention of sudden death. DeMazumder D, Limpitikul WB, Dorante M, et al. Europace. 2016;18(12):1818-1828.
[3] Dynamic analysis of cardiac rhythms for discriminating atrial fibrillation from lethal ventricular arrhythmias. DeMazumder D, Lake DE, Cheng A, et al. Circ Arrhythm Electrophysiol. 2013;6(3):555-561.
Links:
Sudden Cardiac Arrest (National Heart, Lung, and Blood Institute/NIH)
Deeptankar DeMazumder (University of Cincinnati College of Medicine)
DeMazumder Project Information (NIH RePORTER)
NIH Director’s New Innovator Award (Common Fund)
NIH Support: National Heart, Lung, and Blood Institute; Common Fund
Discovering the Brain’s Nightly “Rinse Cycle”
Posted on by Dr. Francis Collins
Getting plenty of deep, restful sleep is essential for our physical and mental health. Now comes word of yet another way that sleep is good for us: it triggers rhythmic waves of blood and cerebrospinal fluid (CSF) that appear to function much like a washing machine’s rinse cycle, which may help to clear the brain of toxic waste on a regular basis.
The video above uses functional magnetic resonance imaging (fMRI) to take you inside a person’s brain to see this newly discovered rinse cycle in action. First, you see a wave of blood flow (red, yellow) that’s closely tied to an underlying slow-wave of electrical activity (not visible). As the blood recedes, CSF (blue) increases and then drops back again. Then, the cycle—lasting about 20 seconds—starts over again.
The findings, published recently in the journal Science, are the first to suggest that the brain’s well-known ebb and flow of blood and electrical activity during sleep may also trigger cleansing waves of blood and CSF. While the experiments were conducted in healthy adults, further study of this phenomenon may help explain why poor sleep or loss of sleep has previously been associated with the spread of toxic proteins and worsening memory loss in people with Alzheimer’s disease.
In the new study, Laura Lewis, Boston University, MA, and her colleagues at the Martinos Center for Biomedical Imaging, Massachusetts General Hospital, Boston. recorded the electrical activity and took fMRI images of the brains of 13 young, healthy adults as they slept. The NIH-funded team also built a computer model to learn more about the fluid dynamics of what goes on in the brain during sleep. And, as it turns out, their sophisticated model predicted exactly what they observed in the brains of living humans: slow waves of electrical activity followed by alternating waves of blood and CSF.
Lewis says her team is now working to come up with even better ways to capture CSF flow in the brain during sleep. Currently, people who volunteer for such experiments have to be able to fall asleep while wearing an electroencephalogram (EEG) cap inside of a noisy MRI machine—no easy feat. The researchers are also recruiting older adults to begin exploring how age-related changes in brain activity during sleep may affect the associated fluid dynamics.
Reference:
[1] Coupled electrophysiological, hemodynamic, and cerebrospinal fluid oscillations in human sleep. Fultz NE, Bonmassar G, Setsompop K, Stickgold RA, Rosen BR, Polimeni JR, Lewis LD. Science. 2019 Nov 1;366(6465):628-631.
Links:
Sleep and Memory (National Institute of Mental Health/NIH)
Sleep Deprivation and Deficiency (National Heart, Lung, and Blood Institute/NIH)
Alzheimer’s Disease and Related Dementias (National Institute on Aging/NIH)
NIH Support: National Institute of Mental Health; National Institute of Biomedical Imaging and Bioengineering; National Institute of Neurological Disorders and Stroke
Early Riser or Night Owl? New Study May Help to Explain the Difference
Posted on by Dr. Francis Collins
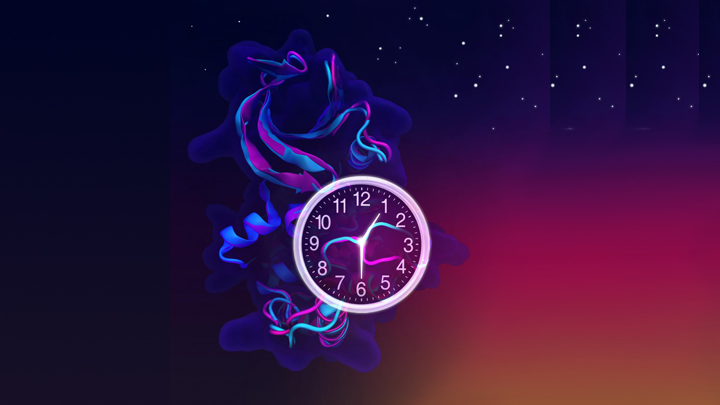
Credit: Clarisse Ricci, University of California, San Diego
Some people are early risers, wide awake at the crack of dawn. Others are night owls who can’t seem to get to bed until well after midnight and prefer to sleep in. Why is this? An NIH-funded team has some new clues based on evidence showing how a molecular “switch” wired into the biological clocks of extreme early risers leads them to operate on a daily cycle of about 20 hours instead of a full 24-hour, or circadian (Latin for “about a day”), cycle [1].
These new atomic-level details, shared from fruit flies to humans, may help to explain how more subtle clock variations predispose people to follow different sleep patterns. They also may lead to new treatments designed to reset the clock in people struggling with sleep disorders, jet lag, or night-shift work.
This work, published recently in the journal eLIFE, comes from Carrie Partch, University of California, Santa Cruz, and her colleagues at Duke-NUS Medical School in Singapore and the University of California, San Diego. It builds on decades of research into biological clocks, which help to control sleeping and waking, rest and activity, fluid balance, body temperature, cardiac rate, oxygen consumption, and even the secretions of endocrine glands.
These clocks, found in cells and tissues throughout the body, are composed of specialized sets of proteins. They interact in specific ways to regulate transcription of about 15 percent of the genome over a 24-hour period. All this interaction helps to align waking hours and other aspects of our physiology to the 24-hour passage of day and night.
In the latest paper, Partch and her colleagues focused on two core clock components: an enzyme known as casein kinase 1 (CK1) and a protein called PERIOD. Clock-altering mutations in CK1 and PERIOD have been known for many years. In fact, CK1 was discovered in studies of golden hamsters more than 20 years ago after researchers noticed one hamster that routinely woke up much earlier than the others [2,3].
It turns out that the timing of biological clocks is strongly influenced by the rise and fall of the PERIOD protein. This daily oscillation normally takes place over 24 hours, but that’s where CK1 enters the picture. The enzyme adjusts PERIOD levels by chemically modifying the protein at one of two sites, thereby adjusting its stability. When one site is modified, it keeps the protein protected and stable. At the other site, it leaves it unprotected and degradable.
Many of these details had been worked out over the years. But, Partch wanted to drill even deeper to answer an essential question: Why does this process normally take 24 hours, which is remarkably slow biochemically? And, what changes in those whose daily cycle gets cut far short?
To find out, her team performed a series of protein structure and biochemical analyses of the CK1 mutation originally found in hamsters, along with several other clock-altering versions of the enzyme found in organisms ranging from flies to humans. What they’ve discovered is a portion of CK1 acts as a switch. When this switch functions normally, it generates a near-perfect 24-hour cycle by keeping PERIOD’s stability just right. In this case, people easily and correctly align their internal clocks to the daily coming and going of daylight.
If the switch favors a faster breakdown of the protein, the daily cycle grows shorter and less tightly bound to daylight. For these early risers, it’s a constant struggle to adjust to life in a 24-hour world. Though they try to get in sync, these early risers are never able to catch up. Conversely, a switch that favors a slower breakdown will lengthen the clock, predisposing some to be night owls.
Such shifts in clock timing can arise from alterations either to the CK1 enzyme or the PERIOD protein. In fact, people with an inherited sleep disorder called Familial Advanced Sleep Phase Syndrome carry a mutation in the PERIOD protein at one of the places that CK1 modifies. The new work shows that this change makes PERIOD more stable by interfering with the enzyme’s ability to mark the protein for degradation.
One thing that makes the CK1 enzyme so fascinating is that it’s extremely ancient. A nearly identical version of the enzyme to the one in humans and hamsters can be found in single-celled green algae! It’s clear that this enzyme and its function in biological clocks is, evolutionarily speaking, rather special. And at one level, that makes total sense—our planet has operated on a 24-hour clock for the entire span of evolutionary time.
The versions of CK1 that Partch’s team studied here are rare in people. She now plans to study other variations that turn up in humans much more often.
Her discoveries are sure to offer a fascinating view on these internal clocks and, pardon the pun, how they make us all tick. She hopes they’ll lead to new ways to adjust the clock in those with sleep disorders and even the means to reset the clock in people who regularly travel overseas or work the night shift.
Ultimately, Partch would like to tap into the crosstalk between biological clocks and the ability of cells to repair their DNA. She wants to see if clock disruptions have any implications for cancer susceptibility. And yes, now’s a good time to find out the answer.
References:
[1] Casein kinase 1 dynamics underlie substrate selectivity and the PER2 circadian phosphoswitch. Philpott JM, Narasimamurthy R, Ricci CG, Freeberg AM, Hunt SR, Yee LE, Pelofsky RS, Tripathi S, Virshup DM, Partch CL. eLIFE. 2020 Feb 11;9.
[2] A mutation of the circadian system in golden hamsters. Ralph MR, Menaker M. Science. 1988 Sep 2;241(4870):1225-7.
[3] Positional syntenic cloning and functional characterization of the mammalian circadian mutation tau. Lowrey PL, Shimomura K, Antoch MP, Yamazaki S, Zemenides PD, Ralph MR, Menaker M, Takahashi JS. Science. 2000 Apr 21;288(5465):483-92.
Links:
Circadian Rhythms (National Institute of General Medical Sciences/NIH)
Advanced Sleep Phase Syndrome, Familial (Genetic and Rare Disease Center/NIH)
Partch Lab (University of California, Santa Cruz)
NIH Support: National Institute of General Medical Sciences; Office of the Director
Anesthesia Study Yields New Insights into Neuroscience of Sleep
Posted on by Dr. Francis Collins

General anesthesia has been around since the 1840s, when most people still traveled by horse and buggy. Yet, in this age of jet planes and electric cars, there are still many unknowns about how general anesthesia works.
The prevailing view has long been that general anesthesia exerts a sedative effect that puts us under, along with a pain-relieving effect that works by temporarily shutting down transmission of sensations from other parts of the body to the brain. Now, researchers have discovered that, at least in mice, some types of general anesthesia may actually activate a specialized area of the brain—findings that not only may provide new insights into anesthesia, but may enhance our understanding of sleep.
In a recent study in the journal Neuron, the NIH-supported lab of Fan Wang at Duke University, Durham, NC, used general anesthesia as a tool to learn more about mammalian brain activity. When they placed mice under multiple classes of general anesthesia, a cluster of neurons were activated in the brain’s hypothalamus that produce slow, oscillating waves similar to those observed in the brains of mice that were sleeping deeply. When these neurons were later artificially deactivated, the effects of general anesthesia were shortened. Experiments in sleeping mice also showed that similar deactivation disrupts natural sleep. The discovery suggests there may be a neural pathway in the mammalian brain that is shared by general anesthesia and natural sleep, perhaps opening the door to new drugs for anesthesia, pain management, and sleep disorders [1].
Specifically, Wang’s group is focused on a part of the hypothalamus called the supraoptic nucleus (SON), which consists of about 3,000 neurons. These neurons are wired into the brain’s neuroendocrine system, a vast regulatory system between brain and body. Each SON neuron has two arms: one extends to the base of the brain, where it triggers the pituitary gland to release hormones; the other directly releases peptide hormones into the general circulation.
It’s not altogether surprising that the hypothalamus would be involved regulating sleep. Previous work had indicated that another part of the hypothalamus might serve as an on-off switch between wakefulness and sleep [2]. The neurons also secrete neuropeptides, such as galanin and GABA. that inhibit areas of the brainstem involved in wakefulness.
But what most fascinated Wang is that her experiments found that SOS cells fire constantly in mice that have been kept awake past their normal bedtime, but stop firing once the animals are allowed to sleep. This prompted her team to turn its attention to the 80 percent of SON neurons that secrete the hormones dynorphin and vasopressin, which are secreted in the general circulation and send a wide range of signals to organs throughout the body.
Though mice are not humans and much more work remains to be done, Wang says her data raise the possibility that sleep, like hunger, may be regulated by a feedback loop of hormones, traveling from brain to other body parts and back. As proposed, the SON cells secrete hormones into the body during periods of wakefulness. As the level of the secreted messengers build up, the body signals to the brain that it’s tired, prompting the SOS neurons to activate a different program, sending signals that tell other parts of the brain to go to sleep.
Discovering a homeostatic sleep mechanism certainly wasn’t what surgeon William T. G. Morton had in mind when he first demonstrated the concept of general anesthesia in the 19th Century. Yet more than 175 years later, Morton’s major clinical advance is now yielding unexpected benefits for basic neuroscience research, providing yet another example of how one never knows where biomedical exploration may take us.
References:
[1] A Common Neuroendocrine Substrate for Diverse General Anesthetics and Sleep. Jiang-Xie LF, Yin L, Zhao S, Prevosto V, Han BX, Dzirasa K, Wang F. Neuron. 2019 Apr 18. pii: S0896-6273(19)30296-X.
[2] Activation of ventrolateral preoptic neurons during sleep. Sherin JE, Shiromani PJ, McCarley RW, Saper CB. Science. 1996 Jan 12;271(5246):216-219.
Links:
Anesthesia (National Institute of General Medical Sciences/NIH)
History of Anesthesia (Wood Library Museum of Anesthesiology, Schaumburg, IL)
Brain Basics: Understanding Sleep (National Institute of Neurological Disorders and Stroke/NIH)
Fan Wang (Duke University School of Medicine, Durham, NC)
NIH Support: National Institute of Mental Health
Sleep Loss Encourages Spread of Toxic Alzheimer’s Protein
Posted on by Dr. Francis Collins

In addition to memory loss and confusion, many people with Alzheimer’s disease have trouble sleeping. Now an NIH-funded team of researchers has evidence that the reverse is also true: a chronic lack of sleep may worsen the disease and its associated memory loss.
The new findings center on a protein called tau, which accumulates in abnormal tangles in the brains of people with Alzheimer’s disease. In the healthy brain, active neurons naturally release some tau during waking hours, but it normally gets cleared away during sleep. Essentially, your brain has a system for taking the garbage out while you’re off in dreamland.
The latest findings in studies of mice and people further suggest that sleep deprivation upsets this balance, allowing more tau to be released, accumulate, and spread in toxic tangles within brain areas important for memory. While more study is needed, the findings suggest that regular and substantial sleep may play an unexpectedly important role in helping to delay or slow down Alzheimer’s disease.
It’s long been recognized that Alzheimer’s disease is associated with the gradual accumulation of beta-amyloid peptides and tau proteins, which form plaques and tangles that are considered hallmarks of the disease. It has only more recently become clear that, while beta-amyloid is an early sign of the disease, tau deposits track more closely with disease progression and a person’s cognitive decline.
Such findings have raised hopes among researchers including David Holtzman, Washington University School of Medicine, St. Louis, that tau-targeting treatments might slow this devastating disease. Though much of the hope has focused on developing the right drugs, some has also focused on sleep and its nightly ability to reset the brain’s metabolic harmony.
In the new study published in Science, Holtzman’s team set out to explore whether tau levels in the brain naturally are tied to the sleep-wake cycle [1]. Earlier studies had shown that tau is released in small amounts by active neurons. But when neurons are chronically activated, more tau gets released. So, do tau levels rise when we’re awake and fall during slumber?
The Holtzman team found that they do. The researchers measured tau levels in brain fluid collected from mice during their normal waking and sleeping hours. (Since mice are nocturnal, they sleep primarily during the day.) The researchers found that tau levels in brain fluid nearly double when the animals are awake. They also found that sleep deprivation caused tau levels in brain fluid to double yet again.
These findings were especially interesting because Holtzman’s team had already made a related finding in people. The team found that healthy adults forced to pull an all-nighter had a 30 percent increase on average in levels of unhealthy beta-amyloid in their cerebrospinal fluid (CSF).
The researchers went back and reanalyzed those same human samples for tau. Sure enough, the tau levels were elevated on average by about 50 percent.
Once tau begins to accumulate in brain tissue, the protein can spread from one brain area to the next along neural connections. So, Holtzman’s team wondered whether a lack of sleep over longer periods also might encourage tau to spread.
To find out, mice engineered to produce human tau fibrils in their brains were made to stay up longer than usual and get less quality sleep over several weeks. Those studies showed that, while less sleep didn’t change the original deposition of tau in the brain, it did lead to a significant increase in tau’s spread. Intriguingly, tau tangles in the animals appeared in the same brain areas affected in people with Alzheimer’s disease.
Another report by Holtzman’s team appearing early last month in Science Translational Medicine found yet another link between tau and poor sleep. That study showed that older people who had more tau tangles in their brains by PET scanning had less slow-wave, deep sleep [2].
Together, these new findings suggest that Alzheimer’s disease and sleep loss are even more intimately intertwined than had been realized. The findings suggest that good sleep habits and/or treatments designed to encourage plenty of high quality Zzzz’s might play an important role in slowing Alzheimer’s disease. On the other hand, poor sleep also might worsen the condition and serve as an early warning sign of Alzheimer’s.
For now, the findings come as an important reminder that all of us should do our best to get a good night’s rest on a regular basis. Sleep deprivation really isn’t a good way to deal with overly busy lives (I’m talking to myself here). It isn’t yet clear if better sleep habits will prevent or delay Alzheimer’s disease, but it surely can’t hurt.
References:
[1] The sleep-wake cycle regulates brain interstitial fluid tau in mice and CSF tau in humans. Holth JK, Fritschi SK, Wang C, Pedersen NP, Cirrito JR, Mahan TE, Finn MB, Manis M, Geerling JC, Fuller PM, Lucey BP, Holtzman DM. Science. 2019 Jan 24.
[2] Reduced non-rapid eye movement sleep is associated with tau pathology in early Alzheimer’s disease. Lucey BP, McCullough A, Landsness EC, Toedebusch CD, McLeland JS, Zaza AM, Fagan AM, McCue L, Xiong C, Morris JC, Benzinger TLS, Holtzman DM. Sci Transl Med. 2019 Jan 9;11(474).
Links:
Alzheimer’s Disease and Related Dementias (National Institute on Aging/NIH)
Accelerating Medicines Partnership: Alzheimer’s Disease (NIH)
Holtzman Lab (Washington University School of Medicine, St. Louis)
NIH Support: National Institute on Aging; National Institute of Neurological Disorders and Stroke; National Center for Advancing Translational Sciences; National Cancer Institute; National Institute of Biomedical Imaging and Bioengineering
Next Page